What is Pseudomonas fluorescens?
Pseudomonas spp. are bacterial species ubiquitous in agricultural soils, well adapted to growing in the rhizosphere and well suited as biocontrol and growth-promoting agents (Weller et al., 2007). Pseudomonas spp. rapidly utilize root and seed exudates, multiply in rhizosphere and spermoshphere environment and in the interior of the plants, produce a wide spectrum of bioactive metabolites (such as antibiotics, siderophores, volatiles, and growth-promoting substances); adapt to environmental stresses and compete aggressively with pathogens (Weller et al., 2007) (Panpatte et al., 2016).
How can Pseudomonas spp. help my crops?
Relief from environmental stresses
Colonies of Pseudomonas spp. are aggressive to persist throughout the growing season, limit the amount of minerals and nutrient availability to pathogens for growth, pre-empt and compete with pathogens for the favored sites on the root, and improve plant growth by suppressing major (root or vascular diseases) and minor (parasites or saprophytes) plant pathogens (Weller et al., 2007). Pseudomonas spp. solubilize phosphorous in soil, release chelating agent called siderophores for iron absorption, nitrogen fixing ability, increase in elements availability to plants to enhance plant growth (Shivasakthi et al., 2014) (Panpatte et al., 2016).
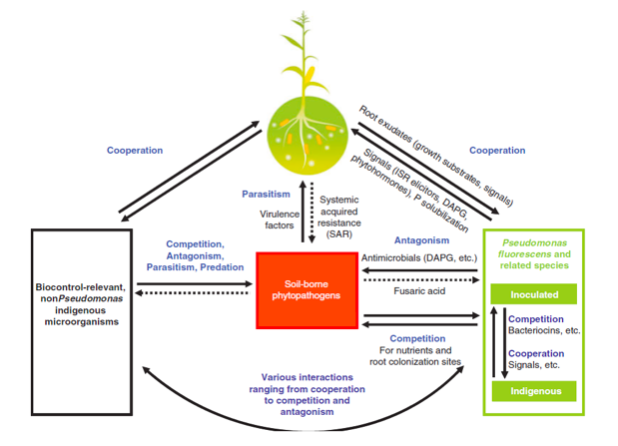
Figure 1: Plant disease management by Pseudomonas spp. as represented by (Couillerot et al., 2008)
Plants immune system response
Pseudomonas spp. demonstrate dynamics of root colonization depicting high microbial diversity, density, synchronized metabolic activity, formidable biological buffering capacity that not only protect from foreign pathogens but also from the introduced pathogens (Weller et al., 2007). During the plant growth application, Pseudomonas spp. produce a potent antibiotic resulting in control and suppression of bacterial disease fire blight even in the areas with streptomycin-resistant populations of the pathogen and provide moderate to excellent efficacy (Couillerot et al., 2008) as represented in figure 1. Pseudomonas spp. produce antimicrobial and metabolites, such as 2,4-diacetylphloroglucinol (DAPG), phenazines, hydrogen cyanide and surfactants, which have received extensive attention and efficacy rate to inhibit various phytopathogens. Pseudomonas spp. also produce extracellular lytic enzymes for protection of plant species and induce an ISR response in plants, and to make plants efficient in fighting back against pathogens (Couillerot et al., 2008).
Plant disease management
The secretion of anti-fungal metabolites by Pseudomonas spp. effectively inhibited mycelial growth by 62-85% and at increased metabolite concentration of 5% completely inhibited the growth of fungal pathogens such as Rhizoctonia bataticola, Fusaricum oxysporum, Xanthomonas oryzae, Rhizoctonia solani (bacterial leaf blight (BB) and sheath blight (ShB) pathogens of rice) (Shivasakthi et al., 2014). Pseudomonas spp. shows high efficacy in controlling the pathogen infections in immature pear fruit, flowers, and whole plants (Pujol et al., 2006). Pseudomonas spp. are also applied in the packinghouses to prevent postharvest fungal disease during storage of citrus, pome, stone fruits, and potatoes (Stockwell et al., 2007).
Should I inoculate my crops with Pseudomonas spp.?
Increased crop yield
The application of Pseudomonas spp. as seed inoculants on crop plants rapidly colonize plant roots, promote growth, enhance crop yield by direct and indirect mechanisms, increase in fresh and dry plant masses resulting in increased yield by 44% (Shivasakthi et al., 2014) (Panpatte et al., 2016). Field experiments on chickpea treated with Bacillus and Pseudomonas spp. delivered maximum significant increase in nodule number, dry weight of nodule, root and shoot and due to additional accumulation of phosphorus, iron, and plant hormones like indole-3-acetic acid (IAA) (Verma et al., 2010).
In presence of Pseudomonas spp. the canola plants delivered increased uptake of phosphorous, improved root (61-65%) and shoot (33-47%) elongation, and promoted plant growth under the gnotobiotic conditions (Lifshitz et al., 1987). In case of soyabean, the Pseudomonas spp. treatment resulted in increased shoot (14-26%) and yield (9-36%) (Schmidt et al., 2015), in alfalfa increased the alginate biosynthesis by 3-fold and decreased the disease infection frequency by 50% (Silo-Suh et al., 2002). In chickpeas increased the biochemical properties of the crop under the soil contaminated with chromium (Oves et al., 2013) and reduction in plant mortality by (63.3-100%) (Negi et al., 2008). Application of Pseudomonas spp. on lentils cultivated in soil depleted with nutrients, resulted in 85% of nodule formation, higher nodule occupancy and delivered increased nitrogen fixation (Sepúlveda-Caamaño et al., 2018) and seed treatment with foliar applications improved yield in lentils (Erdemci et al., 2020).
Will my crop management practice impact Pseudomonas spp. growth?
Crop management practise such as crop rotations, tillage, seed variety, seeding rate, sanitation, summerfallow, trap strips, soil fertility, intercrops, biological control, pest, and insect management etc. (Crop Management, 2020) have little impact on the effectiveness of Pseudomonas spp. season-to season. The Pseudomonas spp. communities are affected long term by plant species and genotypes and through short-term effects during the lifespan of single plant (Schmidt et al., 2015). The current findings on the Pseudomonas spp. are gaining broader incorporation into sustainable agriculture strategies for the crop management against soil pathogens and post-harvest disease (Weller et al., 2007).
Can I use Pseudomonas spp. with other inoculants at the same time?
Pseudomonas spp. can be used simultaneously along with biocontrol inoculants, which posses the property of forming plant growth promoting rhizobacteria (PGPRs) such as Bacillus, Pseudomonas, and Trichoderma (Panpatte et al., 2016). The Pseudomonas spp. plays a major role in PGPRs to deliver benefits across plant growth promotion and suppression of diseases (Negi et al., 2008) and work simultaneously with other species (Bacillus, and Trichoderma) to increase the availability of phosphorus and nitrogen fixation in soil to host plant (Schmidt et al., 2015) (Shivasakthi et al., 2014).
References:
- Pujol, M., Badosa, E., Manceau, C., & Montesinos, E. (2006). Assessment of the environmental fate of the biological control agent of fire blight, Pseudomonas fluorescens EPS62e, on apple by culture and real-time PCR methods. Applied and Environmental Microbiology, 72(4), 2421-2427.
- Weller, D. M. (2007). Pseudomonas biocontrol agents of soilborne pathogens: looking back over 30 years. Phytopathology, 97(2), 250-256.
- Stockwell, V. O., & Stack, J. P. (2007). Using Pseudomonas spp. for integrated biological control. Phytopathology, 97(2), 244-249.
- Couillerot, O., Prigent‐Combaret, C., Caballero‐Mellado, J., & Moënne‐Loccoz, Y. (2009). Pseudomonas fluorescens and closely related fluorescent pseudomonads as biocontrol agents of soil‐borne phytopathogens. Letters in applied microbiology, 48(5), 505-512.
- Sivasakthi, S., Usharani, G., & Saranraj, P. (2014). Biocontrol potentiality of plant growth promoting bacteria (PGPR)-Pseudomonas fluorescens and Bacillus subtilis: a review. African journal of agricultural research, 9(16), 1265-1277.
- Panpatte, D. G., Jhala, Y. K., Shelat, H. N., & Vyas, R. V. (2016). Pseudomonas fluorescens: a promising biocontrol agent and PGPR for sustainable agriculture. In Microbial inoculants in sustainable agricultural productivity (pp. 257-270). Springer, New Delhi.
- Verma, J. P., Yadav, J., & Tiwari, K. N. (2010). Application of Rhizobium sp. BHURC01 and plant growth promoting rhizobactria on nodulation, plant biomass and yields of chickpea (Cicer arietinum L.). International Journal of Agricultural Research, 5(3), 148-156.
- Lifshitz, R., Kloepper, J. W., Kozlowski, M., Simonson, C., Carlson, J., Tipping, E. M., & Zaleska, I. (1987). Growth promotion of canola (rapeseed) seedlings by a strain of Pseudomonas putida under gnotobiotic conditions. Canadian Journal of Microbiology, 33(5), 390-395.
- Sepúlveda-Caamaño, M., Gerding, M., Vargas, M., Moya-Elizondo, E., Oyarzúa, P., & Campos, J. (2018). Lentil (Lens culinaris L.) growth promoting rhizobacteria and their effect on nodulation in co-inoculation with rhizobia. Archives of Agronomy and Soil Science, 64(2), 244-256.
- Erdemci, İ. (2020). Effect of Pseudomonas Fluorescent Rhizobacteria on Growth and Seed Quality in Lentil (Lens Culinaris Medik.). Communications in Soil Science and Plant Analysis, 51(14), 1852-1858.
- Schmidt, J., Messmer, M., & Wilbois, K. P. (2015). Beneficial microorganisms for soybean (Glycine max (L.) Merr), with a focus on low root-zone temperatures. Plant and soil, 397(1-2), 411-445.
- Silo-Suh, L., Suh, S. J., Sokol, P. A., & Ohman, D. E. (2002). A simple alfalfa seedling infection model for Pseudomonas aeruginosa strains associated with cystic fibrosis shows AlgT (sigma-22) and RhlR contribute to pathogenesis. Proceedings of the National Academy of Sciences, 99(24), 15699-15704.
- Oves, M., Khan, M. S., & Zaidi, A. (2013). Chromium reducing and plant growth promoting novel strain Pseudomonas aeruginosa OSG41 enhance chickpea growth in chromium amended soils. European journal of soil biology, 56, 72-83.
- Negi, Y. K., Garg, S. K., & Kumar, J. (2008). Plant growth promoting and biocontrol activities of cold-tolerant Pseudomonas fluorescens isolates against root rot in pea. Indian Phytopathology, 61(4), 461.
- Crop Management, (2020) https://www.saskatchewan.ca/business/agriculture-natural-resources-and-industry/agribusiness-farmers-and-ranchers/crops-and-irrigation/organic-crops/organic-crop-management-insect-management
About Author: VINAYAK PACHAPUR, PhD
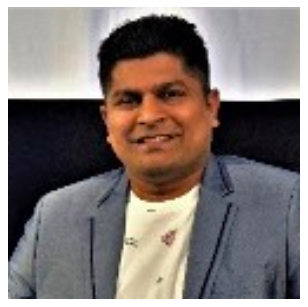
Plant growth mechanism are capable to overcome stress conditions (biotic and abiotic) and develop defense system to resist disease and pathogen occurrence. However, plants need symbiotic and synchronization of beneficial microbes to have stronger growth mechanism and defense system for better yield. To support this approach, Vinayak and CAI team embarked on developing bio-based formulations to boost the consortia between plant and beneficial microbes for better agriculture management and sustainable tool to higher yields.
What is Bacillus spp.?
Bacillus spp. group of bacteria have own acronym as plant growth promoting rhizobacteria (PGPR), form a biofilm on plant root to promote plant growth and provide defensive action to fending off plant pathogens(George H, 2019). The population of B. subtilis, B. licheniformis and B. velezensis are the most common ones and found in healthy plants indicating efficient colonization in rhizosphere (Vinodkumar et al., 2018).
How can Bacillus spp help my crops?
Relief from environmental stresses
Bacillus spp. enhances pigments, hormones (SA, JA, and ABA) and enzymes (catalase, superoxide dismutase, peroxidase, ascorbate peroxidase and glutathione reductase) release to mitigate drought stress and increase water uptake rate. Up or down regulate the antioxidants (CAT, SOD, POD, APX, and GR) and drought responsible genes in host plants. Increase the population of bacterial colony on roots and stimulate root exudation to take up more water against drought damage. Regulate high-affinity transport (HKT1), hydraulic conductivity of roots, stomatal opening, turgor pressure maintenance, osmotic balance and modulate homeostasis (Na/K) to overcome drought stress conditions (Radhakrishnan et al., 2017).
Improved Nutrient efficiency
Bacillus spp. secrete enzymes and organic acids to facilitate the conversion of complex, inorganic phosphate into free, simple, available phosphate for root uptake to improve plant growth metabolisms. Bacillus spp. release ammonia from nitrogenous organic matter, fix atmospheric nitrogen and increase the available form of nitrogen in soil, which enhance plant growth and yield by delaying senescence. The iron-chelating properties of Bacillus spp. solubilize Fe3+ to Fe2+ for easy entry into plant cells to increase nutrient uptake. Induce the synthesis of plant-growth-promoting hormones such as indole-3-acetic acid (IAA), gibberellins, cytokinins, spermidines, to increase root/shoot cell division, elongation and secretion of 1-aminocyclopropane-1-carboxylate (ACC) deaminase to promote plant growth (Radhakrishnan et al., 2017).
Solutions to soils contaminated with heavy metals
Plants treated with Bacillus spp. solubilize, or convert toxic metals to non-toxic forms, reduce the toxic effects of these metals in soil and on plant growth. Enhances heavy metals (Cu, Zn, Cd, Cr and Pb) accumulation and distribution in plants growing in soil contaminated with heavy metals. The association of some Bacillus spp. regulate antioxidants in cells to inhibits oxidative stress damage and triggers plant growth-promoting substances to enable host plants to adapt to metal stress conditions (Radhakrishnan et al., 2017).
Plants immune system response
Bacillus spp. play a role of root colonizer, biocontrol agent, build secure zone, associated mechanism of acquired systemic resistance to increase crop yield under conditions of biotic and abiotic stress (Hashem et al., 2019) (Vinodkumar et al., 2017) as represented in figure 1.
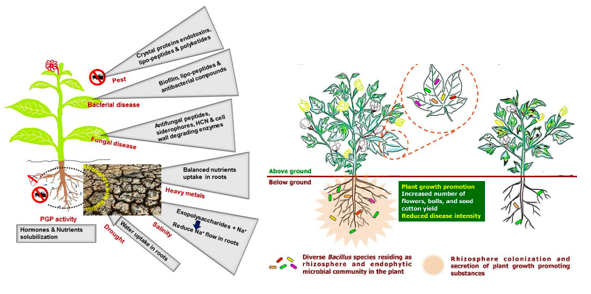
Bacillus spp. activate the immune system known as systemic acquired resistance (SAR) throughout the plant, to ramp-up the natural defence and inhibit soil-borne fungal pathogens (Rhizoctonia, Phytophthora, Fusarium, Botrytis, powdery mildew and Sclerotinia) and bacterial pathogens (Erwinia and Xanthomonas) (George H, 2019) (Radhakrishnan et al., 2017) (Vinodkumar et al., 2017). Various plants viz., carnations, cotton, turmeric, and bananas treated with Bacillus spp. demonstrated minimal percent (4.6%) of Sclerotinia disease incidence and delivered maximum plant growth yield (Vinodkumar et al., 2017) as represented in figure 2.
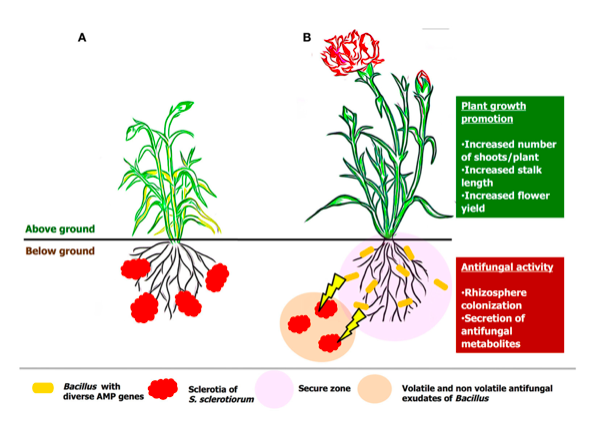
Figure 2: Hypothetical model on the mode of actions of Sclerotina before (A) and after treatment (B) of Bacillus spp. as represented by (Vinodkumar et al., 2017).
Plant disease management
Bacillus spp. exhibits both a direct (synthesis of many secondary metabolites, hormones, cell-wall-degrading enzymes, and antioxidants) and indirect (stimulation of acquired systemic resistance and expression of stress-response genes) biocontrol mechanism to suppress disease caused by pathogens (Vinodkumar et al., 2017). Species of Bacillus produce more than 24 types of antibiotics, antimicrobial metabolites, and other toxin compounds such as lipopeptides, Iturin A, fencygins, surfactin, macrolactin and bacillomycin etc. Lipopeptides interact with fungal cell membranes to create holes and to kill the fungal in the process. Iturin A and fencygins exhibit antifungal and antibacterial activity to inhibit spore generation and increased cell membrane permeability with formation of ion-conducting pores to damage and cause pest larval death(George H, 2019) (Radhakrishnan et al., 2017) (Vinodkumar et al., 2017).
In case of diseased plant, the pathogenic bacteria affect the defence system by decreasing the enzyme activity (phenylalanine ammonia-lyase (PAL), lipoxygenase etc.). However, with the application of Bacillus spp. these defence enzyme and antioxidant enzymes activities are accelerated, triggering the biosynthesis of polyphenol compounds (lignin, flavonoids and phenylpropanoids) to develop plant resistance against environmental stimuli(Radhakrishnan et al., 2017). Bacillus spp. release antiviral compounds to fight against viral pathogens, considered as second largest group of plant disease after fungi. In addition, trigger induced systemic resistance (ISR), biofilm formation, surfactin production and increase the expression of disease-resistant signaling genes, defence genes and cell wall expansion genes in plants.
Altogether, inhibit viral coat protein synthesis, prevent viral damage, and provide enhanced plant growth against cucumber and tobacco mosaic virus infection in plants. In case of root-knot nematodes, Bacillus spp. prevent the infections of nematode populations, inhibit the growth, develop resistance by reducing gall, egg masses in plants and release crystal proteins responsible for nematicidal activity against nematodes(Radhakrishnan et al., 2017). Exploitation of Bacillus spp. with multifaceted action and with diverse anti-microbial genes will together serve an alternative method for the management and control of fungal, bacterial, and viral diseases in crop plants.
Should I inoculate my crops with Bacillus spp.?
Increased crop yield
The application of Bacillus spp. on soybean was carried out in a conventional system under sprinkler irrigation, resulted in increased yield (15-25%) due to increased accumulation of storage proteins and seed vigor (Tavanti et al., 2020), decreased root rot occurrence by (43-63%), increased plant height (9-18%), root dry weight (8-19%) and altogether suggesting effective alternatives to fungicides (Zhang et al., 2009). In case of cowpea and mash bean plants, the Bacillus spp. application as seed dressing and soil drenching resulted in significant increase in germination (70-100%), length (2-7%) and weight (2-5%) of shoot and root and controlled the infection of soil borne root-infecting fungi (Dawar et al., 2010). The disease management of vascular wilt on lentils using Bacillus spp. delivered highest inhibition of 36%, improved growth and increased dry weight of lentil plants (El‐Hassan et al., 2006). The effect of Bacillus spp. on canola plant reduced clubroot severity by 62-83% due to upregulation of defense response gene by 2.2-23-fold relative to control plants (Lahlali et al., 2013) and in small-scale trial significantly controlled the damping-off and occurrence of fungal disease on alfalfa seeds (Handelsman et al., 1990).
Will my crop management practice impact Bacillus spp growth?
Crop management practise such as crop rotations, tillage, seed variety, seeding rate, sanitation, summer-fallow, trap strips, soil fertility, intercrops, biological control, pest, and insect management etc. (Crop Management, 2020) have little impact on the effectiveness of AMF season-to season. The application of Bacillus as PGPRs introduced in agricultural systems will increase the resistance towards biotic and abiotic stresses to produce sustainable, environmentally-friendly management tools.
Can I use Bacillus spp. with other inoculants at the same time?
Bacillus spp. can be used simultaneously along with biocontrol inoculants, which posses the property of forming plant growth promoting rhizobacteria (PGPRs) such as Bacillus, Pseudomonas, and Trichoderma (Panpatte et al., 2016). The Bacillus spp. plays a major role in PGPRs to deliver benefits across plant growth promotion and suppression of diseases (Negi et al., 2008) and work simultaneously with other species (Pseudomonas, and Trichoderma) to increase the availability of phosphorus and nitrogen fixation in soil to host plant (Schmidt et al., 2015) (Shivasakthi et al., 2014). Soils inoculated with the combination of Bacillus spp. and Trichoderma improved the efficacy of an antagonists and reduced the occurrence of infections to seeds, this effect increased with increased dose (Dawar et al., 2010) and consortial application with other beneficial microbes resulted in reduced cotton leaf curl occurrence and resistance against cucumber mosaic virus (Vinodkumar et al., 2018).
References:
- George H, (2019) Controlling plant pathogens with the biofungicide Bacillus subtilis, link: https://gardenerspath.com/how-to/organic/bacillus-subtilis/
- Radhakrishnan, R., Hashem, A., & Abd_Allah, E. F. (2017). Bacillus: a biological tool for crop improvement through bio-molecular changes in adverse environments. Frontiers in physiology, 8, 667.
- Hashem, A., Tabassum, B., & Abd_Allah, E. F. (2019). Bacillus subtilis: A plant-growth promoting rhizobacterium that also impacts biotic stress. Saudi journal of biological sciences, 26(6), 1291-1297.
- Vinodkumar, S., Nakkeeran, S., Renukadevi, P., & Malathi, V. G. (2017). Biocontrol potentials of antimicrobial peptide producing Bacillus species: multifaceted antagonists for the management of stem rot of carnation caused by Sclerotinia sclerotiorum. Frontiers in microbiology, 8, 446.
- Vinodkumar, S., Nakkeeran, S., Renukadevi, P., & Mohankumar, S. (2018). Diversity and antiviral potential of rhizospheric and endophytic Bacillus species and phyto-antiviral principles against tobacco streak virus in cotton. Agriculture, Ecosystems & Environment, 267, 42-51.
- Tavanti, T. R., Tavanti, R. F., Galindo, F. S., Simões, I., Dameto, L. S., & Sá, M. E. D. (2020). Yield and quality of soybean seeds inoculated with Bacillus subtilis strains. Revista Brasileira de Engenharia Agrícola e Ambiental, 24(1), 65-71.
- Zhang, J. X., Xue, A. G., & Tambong, J. T. (2009). Evaluation of seed and soil treatments with novel Bacillus subtilis strains for control of soybean root rot caused by Fusarium oxysporum and F. graminearum. Plant disease, 93(12), 1317-1323.
- Dawar, S., Wahab, S., Tariq, M., & Zaki, M. J. (2010). Application of Bacillus species in the control of root rot diseases of crop plants. Archives of Phytopathology and Plant Protection, 43(4), 412-418.
- El‐Hassan, S. A., & Gowen, S. R. (2006). Formulation and delivery of the bacterial antagonist Bacillus subtilis for management of lentil vascular wilt caused by Fusarium oxysporum f. sp. lentis. Journal of Phytopathology, 154(3), 148-155.
- Lahlali, R., Peng, G., Gossen, B. D., McGregor, L., Yu, F. Q., Hynes, R. K., … & Boyetchko, S. M. (2013). Evidence that the biofungicide Serenade (Bacillus subtilis) suppresses clubroot on canola via antibiosis and induced host resistance. Phytopathology, 103(3), 245-254.
- Handelsman, J., Raffel, S., Mester, E. H., Wunderlich, L., & Grau, C. R. (1990). Biological control of damping-off of alfalfa seedlings with Bacillus cereus UW85. Applied and environmental microbiology, 56(3), 713-718.
- Crop Management, (2020) https://www.saskatchewan.ca/business/agriculture-natural-resources-and-industry/agribusiness-farmers-and-ranchers/crops-and-irrigation/organic-crops/organic-crop-management-insect-management.
- Schmidt, J., Messmer, M., & Wilbois, K. P. (2015). Beneficial microorganisms for soybean (Glycine max (L.) Merr), with a focus on low root-zone temperatures. Plant and soil, 397(1-2), 411-445.
- Sivasakthi, S., Usharani, G., & Saranraj, P. (2014). Biocontrol potentiality of plant growth promoting bacteria (PGPR)-Pseudomonas fluorescens and Bacillus subtilis: a review. African journal of agricultural research, 9(16), 1265-1277.
What is Trichoderma spp.?
Trichoderma spp. are genus of fungi commonly found in endophytic plant symbionts, grows along the outermost root surface, and feeds on soil microbes attracted to the root system. This fungus grows with root intercellular spaces, coordinates plant defense system and is widely used to control disease, enhance plant growth, and improve the crop yield (Siemering et al., 2016).
How can Trichoderma spp. help my crops?
Relief from environmental stresses
In case of seed treatment, Trichoderma spp. releases metabolite (gliotoxin) that mimic plant growth hormone, enhances seed generation percentage, activate enzymes and phytohormones property to alter soil microflora for increased nutrient accessibility and uptake-rate in soil. Improve plant morphology and physiology with root enhancement, alter photosynthetic rate during the early stage of plant growth, control the environmental buffering (pH, drought, water-logging, cold and heat) and involved in phosphorus uptake, cellulase release to organic matter decomposition, mineral chelation and siderophore production (Singh et al., 2018).
Biotic and Abiotic conditions
Treated seed, germinate consistently faster and more uniformly in comparison to untreated seeds under biotic stress (seed and seedling disease caused by Pythium ultimum) and abiotic stresses (osmotic, salinity, chilling, or heat stress). The plant–fungus association reduces the accumulation of toxic reactive oxygen species (ROS) and lipid peroxides in seedlings under stress conditions. Improves seedling vigor and ameliorates stress by inducing physiological protection in plants against oxidative damage (Mastouri et al., 2010) (Zin et al., 2016) as represented in figure 1.
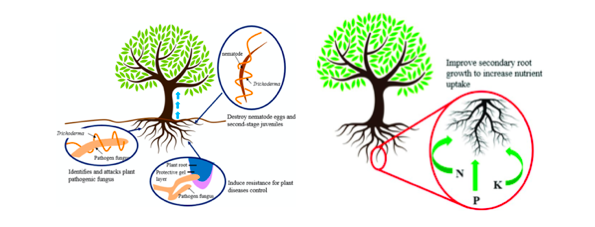
Plants immune system response
Trichoderma-pathogen interactionfollows different mode of action. In case of mycoparasitism involves direct attack on pathogen, which involves recognition of the host: by carbohydrate binding with pathogens, coiling and appressoria formation: induce penetration, secretion of hydrolytic enzymes: fungitoxic cell-wall-degrading enzymes, penetrations of the hyphae: dissolution of the cell walls, and finally lysis of the host: direct entry and killing of the pathogen.
In case of antibiosis mode of action, secondary metabolites, or antibiotics (gliovirin, gliotoxin, viridin, viridol, koninginins, pyrones and peptaibols) produced are detrimental for the growth of pathogens(Singh et al., 2018). These metabolites inhibit the growth of soil-borne plant pathogens, prevents spore germination, and induce extensive apoptotic programmed cell death. During competition mode of action, in the vicinity and molecular assembly of Trichoderma spp. restrict the accessibility of limiting nutrients and starvation of nutrients leads to the death of pathogens. (Singh et al., 2018).
Plant disease management
Trichoderma spp. plays an antagonistic role against the growth of clubroot pathogen (Plasmodiophora brassicae), causes disease reduction (Botero Ramírez et al., 2016), by completely suppressing formation of galls. In addition, enhances photosynthesis with increased chlorophyll activity followed with improved nutrition uptake correlated with higher yields in agricultural and horticultural crops (Suada et al., 2017) (Upadhyay et al., 2020) as represented in figure 2. Similar role against soil-borne fungal pathogens such as Sclerotium rolfsi and Rhizoctonia solani, showed systematic resistance response and reduction in severity of the diseases up to 80% (Debao et al., 1993) (Cheah et al., 2000) (Hanson et al., 2000).
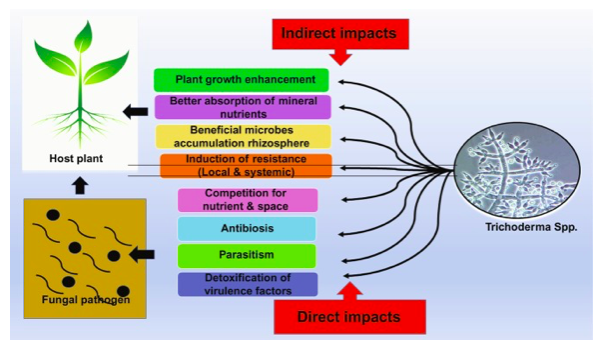
Figure 2: Plant disease management by Trichoderma spp. as represented by (Upadhyay et al., 2020).
Should I inoculate my crops with Trichoderma spp.?
Increased crop yield
Trichoderma spp. account for 60% of the registered bio-fungicides in the market and are the most successful biocontrol agents. Trichoderma spp. possess many qualities such as improved seed germination with plant growth development, increase nutrient uptake rate in plants alter morphological and physiological features, enhance nitrogen-use efficiency among different crops, and assist photosynthetic efficiency(Sood et al., 2020). Guaranteed high yield production in case of crops like mustard, wheat, corn, tuberose, sugarcane, tomato, okra, etc. In legume crops such as lentil and pea, Trichoderma spp. treatment delivered resistance to root, promotion in plant root and increase in shoot biomass was observed (Bazghaleh et al., 2020), showed significant positive effects on seed germination, root length and vigour index in soybean (Živanov et al., 2013), similar effect in rice and potato (Abbas et al., 2017). During growth of pea plants, showed increased percentage of germination rate, increased photosynthetic pigment (chlorophyll), carotenoid, total sugar, and protein content (Singh et al., 2015). In case of lentil, delivered lowest foot and root rot incidence (6.9%), highest seed germination (82.08%), maximum plant stand (93.12%) and the highest seed yield (3726.67 kg ha1) (Kashem et al., 2011) and improved tolerance towards salinity and drought for rapeseed plant (Poveda et al., 2020).
Will my crop management practice impact Trichoderma spp. growth?
Crop management practise such as crop rotations, tillage, seed variety, seeding rate, sanitation, summerfallow, trap strips, soil fertility, intercrops, biological control, pest, and insect management etc. (Crop Management, 2020) have little impact on the effectiveness of Trichoderma spp. season-to season. Tillage practices have no impact on Trichoderma spp. colonization, they are not crop specific and colonize all crop irrespective of crop rotations and seed treatment is more effective in providing plant benefits (Siemering et al., 2016).
Can I use Trichoderma spp. and Arbuscular Mycoorhizal Fungi (AMF) inoculants at the same time?
Few research data suggest naturally co-existence of AMF and Trichoderma spp. in rhizosphere, work simultaneously during co-inoculation for increased crop yield by 37%. However, differ in concentrations of the inoculants may temporarily upset the work and benefit balance on the host plants (Siemering et al., 2016). The application of Trichoderma spp. favours the access of AMF to non-host rapeseed roots to produce significant increase in colonization, promote growth and stimulate systemic defense (Poveda et al., 2019). Work simultaneously with other species (Bacillus, and Trichoderma) to increase the availability of phosphorus and nitrogen fixation in soil to host plant (Schmidt et al., 2015) (Shivasakthi et al., 2014).
<< See Part 1 Continue to Part 3 >>
References:
- Mastouri, F., Björkman, T., & Harman, G. E. (2010). Seed treatment with Trichoderma harzianum alleviates biotic, abiotic, and physiological stresses in germinating seeds and seedlings. Phytopathology, 100(11), 1213-1221.
- Zin, N. A., & Badaluddin, N. A. (2020). Biological functions of Trichoderma spp. for agriculture applications. Annals of Agricultural Sciences, 65(2), 168-178.
- Botero Ramírez, A. (2016). Effect of three Trichoderma species on clubroot disease in cabbage. Escuela de Posgrados. Universidad Nacional de Colombia, Masters Thesis.
- Suada, I. K. (2017). The potential of various indigenous Trichoderma spp. to suppress Plasmodiophora brassicae the pathogen of clubroot disease on cabbage. Biodiversitas Journal of Biological Diversity, 18(4), 1424-1429.
- Debao, X. T. Z. J. L. (1993). Antagonism of Trichoderma harzianum T82 and Trichoderma sp NF9 against soil-borne fungous pathogens [J]. Acta Phytopathologica Sinica, 1.
- Cheah, L. H., Veerakone, S., & Kent, G. (2000). Biological control of clubroot on cauliflower with Trichoderma and Streptomyces spp. New Zealand Plant Protection, 53, 18-21
- Upadhyay H., Kumar V., Kumar S. (2020). Chapter 6 – The effect of fungal metabolites on soil-borne pathogenic fungi. https://doi.org/10.1016/B978-0-12-821007-9.00006-1
- Singh, A., Shukla, N., Kabadwal, B. C., Tewari, A. K., & Kumar, J. (2018). Review on plant-Trichoderma-pathogen interaction. Int J Curr Microbiol Appl Sci, 7(2), 2382-2397.
- Sood, M., Kapoor, D., Kumar, V., Sheteiwy, M. S., Ramakrishnan, M., Landi, M., … & Sharma, A. (2020). Trichoderma: The “Secrets” of a Multitalented Biocontrol Agent. Plants, 9(6), 762.
- Abbas, A., Jiang, D., & Fu, Y. (2017). Trichoderma spp. as antagonist of Rhizoctonia solani. Journal of Plant Pathology and Microbiology, 8(3).
- Poveda, J. (2020). Trichoderma parareesei favors the tolerance of rapeseed (Brassica napus L.) to salinity and drought due to a chorismate mutase. Agronomy, 10(1), 118.
- Bazghaleh, N., Prashar, P., Woo, S., & Vandenberg, A. (2020). Effects of Lentil Genotype on the Colonization of Beneficial Trichoderma Species and Biocontrol of Aphanomyces Root Rot. Microorganisms, 8(9), 1290.
- Tančić, S., Skrobonja, J., Lalošević, M., Jevtić, R., & Vidić, M. (2013). Impact of Trichoderma spp. on soybean seed germination and potential antagonistic effect on Sclerotinia sclerotiorum. Pesticidi i fitomedicina, 28(3), 181-185.
- Singh, B. N., Singh, A., Singh, G. S., & Dwivedi, P. (2015). Potential role of Trichoderma asperellum T42 strain in growth of pea plant for sustainable agriculture. J. Pure Appl. Microbiol, 9(2), 1069-1074.
- Kashem, M. A., Hossain, I., & Hasna, M. K. (2011). Use of Trichoderma in biological control of foot and root rot of lentil (Lens culinaris Medik). Int. J. Sustain. Crop Prod, 6(1), 29-35.
- Poveda, J., Hermosa, R., Monte, E., & Nicolás, C. (2019). Trichoderma harzianum favours the access of arbuscular mycorrhizal fungi to non-host Brassicaceae roots and increases plant productivity. Scientific reports, 9(1), 1-11.
- Siemering, G., Ruark, M., & Geven, A. (2016). The value of Trichoderma for crop production. University of Wisconsin–Extension, Cooperative Extension.
- Crop Management, (2020) https://www.saskatchewan.ca/business/agriculture-natural-resources-and-industry/agribusiness-farmers-and-ranchers/crops-and-irrigation/organic-crops/organic-crop-management-insect-management.
- Schmidt, J., Messmer, M., & Wilbois, K. P. (2015). Beneficial microorganisms for soybean (Glycine max (L.) Merr), with a focus on low root-zone temperatures. Plant and soil, 397(1-2), 411-445.
- Sivasakthi, S., Usharani, G., & Saranraj, P. (2014). Biocontrol potentiality of plant growth promoting bacteria (PGPR)-Pseudomonas fluorescens and Bacillus subtilis: a review. African journal of agricultural research, 9(16), 1265-1277.